Introduction
- The human gut is colonized by microbiota early in life.
- It is now recognized that bacteria in the gut have many beneficial function and play a key role in immune system development and protection against pathogens.
- At birth, the infant gut is sterile, and becomes colonized with bacteria during delivery.
- Thereafter, the microbial content of the intestine is determined by environmental exposure and host genetics.
- Post-partum, diet is arguably the greatest environmental determinant of the intestinal microbiota with high variability within the gut microbiome during the first year of life.
- Significant differences have also been noted in the microbial composition of the adult human intestine across cultures with the prototypic Western diet known to be low in fibre predominated by Firmicutes and low in Bacteroidetes, more common in the high-fibre diets of developing countries.
- The intestinal microbiome makeup thus is host-specific, dynamic, and sensitive to dietary influence, particularly in infancy.
Microbiota and Immune Homeostasis.
- Intestinal microbiota are increasingly recognized for their role in regulating immune cell homeostatis.
- Signals from intestinal bacteria are known for priming systemic immune responses and for regulating pro- and anti-inflammatory host immune responses
- These include T regulatory cells (Tregs) and T helper 17 (Th17) cells
- These interconnections between intestinal tissue and microbiota are delicate and can be easily disrupted
- The consequences of disruption can include inflammation and sepsis.
- Treg differentiation in the mouse model is facilitated in the gut by commensal organisms such as fragilis and Clostridial species.
- Furthermore, segmented filamentous bacteria (SFB) are responsible for the induction of intestinal Th17 cytokines in mice.
- As Th17 cells play an important role in host defense against invading bacterial pathogens, alterations in the composition of these cells could lead to increased disease susceptibility.
- Intestinal bacteria also influence the fate of intestinal epithelial cells (IEC).
- In both germ-free and antibiotic-treated mice, who have an absence of bacteria, IEC have lower expression of bacterial pattern recognition receptors which increases disease susceptibility
- Large shifts in microbial composition can lead to community imbalance, a state known as dysbiosis.
Innate Epithelial Barrier Defense
- It is of paramount importance to host health that the intestinal microbiota is kept at a distance from IECs, minimizing the likelihood of tissue damage and invasion
- Innate immune strategies include the use of a mucus layer, antimicrobial peptides (AMPs) and innate lymphoid cells (ILCs) functioning in concert to confine much of the community to the lumen of the intestinal tract
- The strategies used are different in the large intestine and the small intestine but ultimately work to promote mutualistic interactions and anatomical containment of the microbiota (Figure 1)
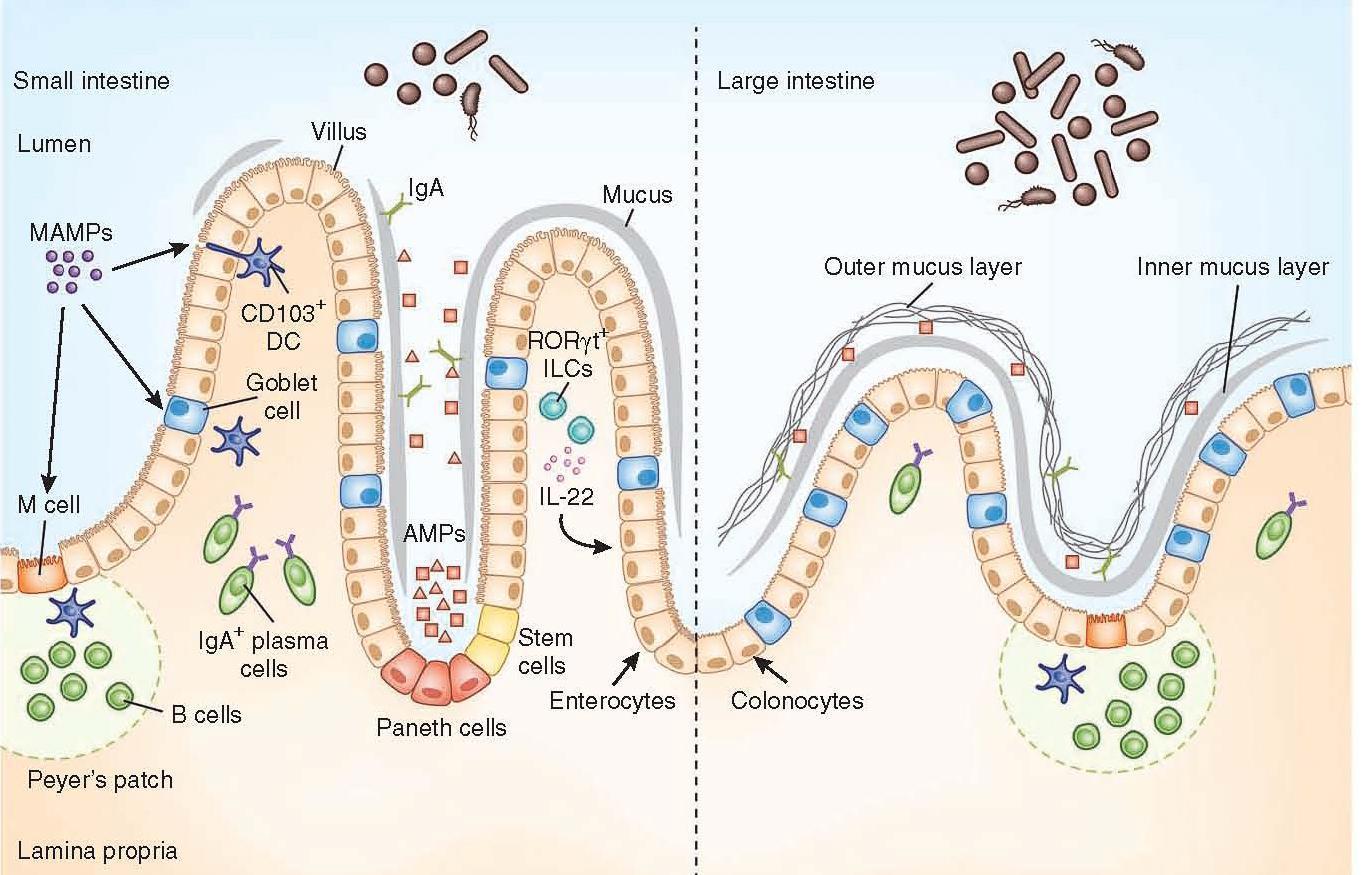
Figure 1: Anatomical containment of the microbiota along the intestine. The intestinal epithelium comprises a single layer of enterocytes or colonocytes, and it is the role of the immune system to protect the integrity of this barrier. In the small intestine, absorptive requirements for enterocytes results in a discontinuous mucus layer, with fewer goblet cells. Here Paneth cells are enriched in the crypts, secreting AMPs, which can cross-link with the mucus layer. Through this barrier, sampling of MAMPs can be mediated through antigen uptake by M cells and goblet cells to dendritic cells (DCs), along with direct trans-epithelial luminal sampling from DCs. RORγt ILCs can sense microbial signals and produce IL-22 to aid in IEC barrier function. Commensal-specific IgA is produced by plasma cells in the lamina propria, mediated by DCs in a T cell–independent mechanism. The large intestine uses a thick, continuous mucus layer to compartmentalize the microbiota, with IgA and AMPs having a secondary role. [Brown E, Sadarangani M, & B Finlay B. The role of the immune system in governing host-microbe interactions in the intestine. Nature Immunology 2013; 14(7): 660-667]
- The mucus layer is the vital component of the innate immune system, segregating the microbiota from the intestinal epithelium.
- Specialized epithelial cells called goblet cells secrete mucin glycoproteins (mucus)
- The thickness of the inner mucus layer is an important physiological feature and has a role in susceptibility to pathogen-induced and commensal-induced inflammation
- The outer mucus layer has a role in shaping the mucosa-associated microbiota by providing glycans as a nutrient source
- The density of the inner layer also limits the direct contact of these bacteria with epithelial cells
- The mucus layer in the small intestine lacks distinct inner and outer layers, and is discontinuously secreted along the apical surface
- Consequently, vast arsenals of AMPs take on a larger role in segregating the microbiota from the epithelium
- Paneth cells are essential to containing the microbiota, and a loss of Paneth cells results in increased invasion of the epithelial barrier by pathogenic and symbiotic microbes
Innate Immune Barriers
- Innate barriers ensure a tolerant response to the microbiota.
- The presence of a functional barrier, with normal amounts of PRRs, mucus, AMPs and secreted IgA, promotes intestinal homeostasis with the microbiota.
- The microbiota is segregated away from the IECs, and the intestinal immune system directs a largely tolerant response to the resident commensals.
- MAMPs stimulate the epithelial secretion of IL-33, TGF-β, TSLP, BAFF and APRIL, all promoting the development of tolerogenic immune cell responses to the microbiota.
- This cytokine environment enriches for CD103+ dendritic cells (DCs), which aid in the development of Treg cells secreting IL-10 and TGF-β.
- Treg cells and CD103+ DCs stimulate the production of commensal- specific IgA.
- Barrier integrity of the IECs is enhanced by secretion of IL-22 by RORγt ILCs in this environment.
- In immunodeficiency or inflammatory syndromes with an innate barrier defect (for example, IBD, CVID or HIV infection), the intestinal immune system directs a potentially harmful pro-inflammatory response to the microbiota to clear invading bacteria and dysbiosis occurs.
- In this environment, the epithelium can secrete IL-1 and IL-6 in response to danger signals.
- Secretion of IL-12 and IL-23 by DCs and macrophages promote a TH1 and TH17 response.
- These T helper cells secrete high levels of IFN-γ and IL-17A, respectively, and T-bet+ ILCs also accumulate to produce IFN-γ.
- A breach in the epithelial barrier by the microbiota in this situation can also lead to higher levels of B cells secreting commensal-specific IgG.
- Figure 2 shows how these different immune components fit together
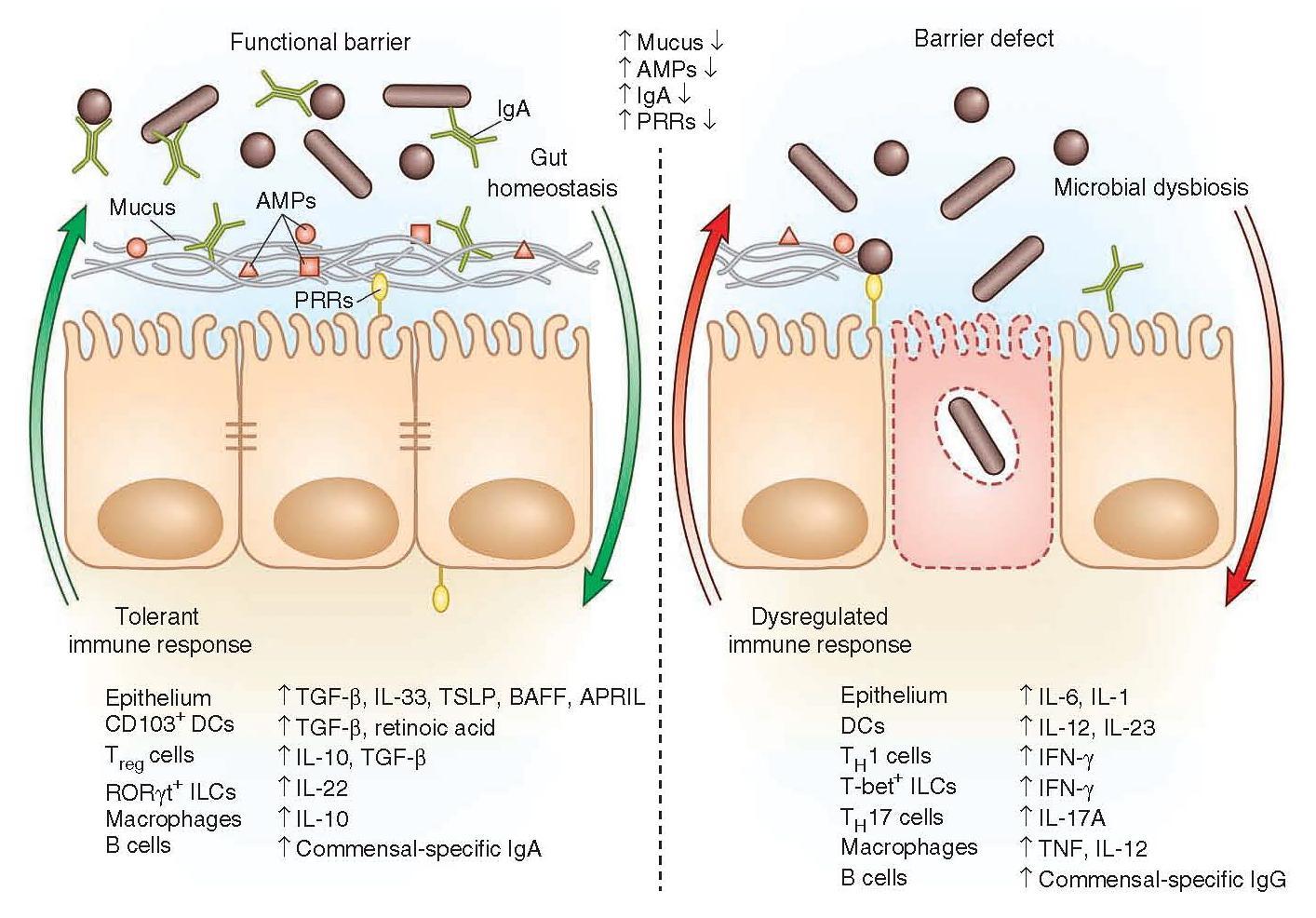
Figure 2: Immune factors that are involved in a functional barrier in the intestinal immune tract and allowing the co-existence of commensal bacteria, contrasting with a barrier defect leading to dysbiosis and dysregulated immune responses. [Brown E, Sadarangani M, & B Finlay B. The role of the immune system in governing host-microbe interactions in the intestine. Nature Immunology 2013; 14(7): 660-667]
Treg Cells and the Microbiome
- Treg cells are thought to be regulated by the microbiota.
- From mouse studies, there is a larger proportion of Treg cells among the CD4+ T cell population in the large intestine and, to a lesser degree, in the small intestine than found in lymph nodes or other secondary lymphoid organs.
- Removal of microbial flora (with antibiotics) results in an increased fraction of Treg cells in the small intestine, although there are fewer total CD4+ T cells and Th17 cells are absent.
- In the large intestine, in contrast, there is a substantial reduction in Treg cell numbers in germ-free mice.
- This reduction is largely in the iTreg population, which is defined by the absence of expression of the transcription factor Helios.
- The iTreg cells were largely restored by colonization of germfree mice with a mixture of Clostridial strains
- Colonization with the mixed Clostridia was also sufficient to induce IL-10, an anti-inflammatory cytokine, and CTLA4, a molecule that inhibits T cell activation, in a substantial fraction of the iTreg cells.
- When neonates kept in a conventional specific pathogen-free facility were gavaged with the Clostridia, the animals were protected from inflammation induced with disodium sulfate (DSS) or oxazalone and displayed lower levels of ovalbumininduced IgE.
- Moreover, Clostridia elevated the proportion of Treg cells not only in the gut, but also in other organs, including lung, liver, and skin.
- One possibility for the increased numbers of iTreg cells in response to Clostridia is that a) these cells have a unique TCR repertoire that confers specificity for commensal bacteria, and/or b) that bacterial products can influence the Th17:Treg balance, potentially by favoring iTreg cell differentiation or expansion
- The induction of iTreg is therefore a mechanism whereby tolerance to specific commensal bacteria is favoured
Innate Lymphoid Cells (ILCs) and the Microbiome
- As discussed in other sections, adaptive immune responses regulated by the selection of T and B cell receptor repertoires
- There is cognate antigen (Ag)–peptide–MHC recognition of Ag-specific T helper (TH) cells, which results in proliferation and differentiation into effector TH cell subsets with distinguishable cytokine profiles (see sections 2 and 5).
- The regulation of commitment, expansion and contraction of these responses is achieved, in part, by transcriptional networks
- As described in the earlier sections, differing subsets of Th cells expand and contract, such as Th1, Th2, Th9, Th17 and Tregs.
- A relatively new subset of cells have been described, which emerge as a first line of defense at mucosal barriers – innate lymphoid cells (ILCs).
- ILCs belong to the lymphoid lineage and develop from common lymphoid progenitor cells, but unlike T and B cells, they lack rearranged Ag-receptors.
- ILCs are found in not only mucosal tissue (intestine and lungs), but also skin, liver and fat
- The cells depend on the expression of the common cytokine recep-tor γ chain (γc chain) and the transcriptional repressor inhibitor of DNA binding 2 (ID2) for their development
- ILCs are classified into three groups, which are able to immediately react to microbial and inflammatory challenge with cytokine production and so limit pathogen spread and tissue injury
- Group 1 ILCs consist of cNK cells and so-called helper ILC1s; both secrete the TH1-type cytokine IFN-γ.
- Group 2 ILCs are characterized by the production of TH2-type cytokines IL-4, IL-5, and/or IL-13.
- Group 3 ILCs include fetal lymphoid tissue-inducer (LTi) cells, as well as adult ILC3s either expressing the natural cytotoxicity receptor (NCR) NKp46 (NCR+ILC3s) or lacking this molecule (NCR− ILC3s).
- There is also a certain level of plasticity between the ILC groupings, and should not be considered rigid
- For example, ILCs have the potential to modulate their phenotypic and transcriptional signature upon activation and inflammation and may switch functions.
- ILCs can sense environmental and inflammatory signals rapidly, and respond with the secretion of cytokines important for immune defense, allergic reactions, and tissue repair
- Group 3 ILCs are relevant for protection of mucosal tissues and the ILC3 subset depend on the transcription factor RORγt for their development and produce IL-22 (a Th17-type cytokine).
- Figure 3 shows the interactive nature of ILC3 with CD4+ T cells, DC’s, intestinal epithelium and commensal bacteria in both the intestine and the spleen
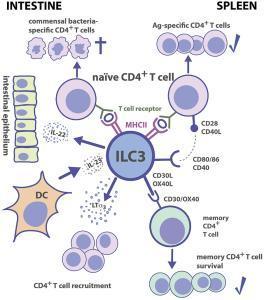
Figure 3: Group 3 ILC-CD4+ T cell interactions. Tissue localization greatly affects the outcome of Ag-dependent T cell-ILC3 interaction. Intestinal ILC3s maintain tolerance toward commensal microbiota, while splenic ILC3s are efficient in the induction of Ag-specific CD4+ T cell responses and memory CD4+ T cell survival. [von Burg N, Turchinovich G and Finke D (2015) Maintenance of immune homeostasis through ILC/T cell interactions. Front. Immunol. 6:416]
- IL-22 protects IECs from bacterial infections and in promotion of tissue repair and it has been shown in mice that ILC3s are important for protection from gut pathogen infection
- ID2-dependent ILC3s produce IL-22 and limit early colonization of pathogen through homeostasis of the microbiota (Figure 4)
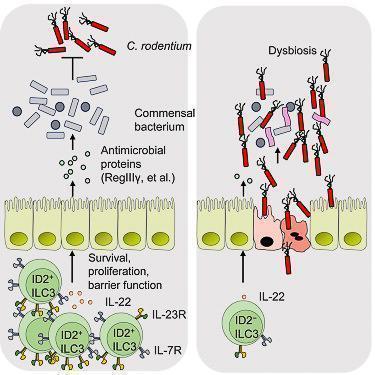
Figure 4: ID2 is essential to mediate colonization resistance against C. rodentium and continued expression of ID2 is required for the homeostasis of ILC3s. Lack of ID2-dependent ILC3s results in loss of immune homeostasis and gut dysbiosis. [Guo X et al Innate Lymphoid Cells Control Early Colonization Resistance against Intestinal Pathogens through ID2-Dependent Regulation of the Microbiota. Immunity 2015; 42:731-43]
The Microbiome and Disease Susceptibility
- Critical to the maintenance of immune homeostasis is where, when, and how immune responses prevent tissue injury.
- The intestine has been extensively studied with respect to cellular networks and pathways patrolling tissue integrity and regulating inflammation.
- Treg and TH17 cells are the most abundant CD4+ TH cells in the intestinal mucosa under steady state.
- The balance between the two subsets is crucial for the outcome of mucosal immune responses
- ILCs are important “early sentinel” cells, which connect innate and adaptive immunity by sensing environmental changes, such as infections and inflammation and determine the balance of Th/Treg cells
- Altered intestinal microbiota has long been associated with inflammatory disorders of the GI tract, including inflammatory bowel disease (IBD).
- Likewise, a number of systemic diseases such as diabetes and rheumatoid arthritis have been associated with altered intestinal microbiota.
- The composition of the gut microbiota may influence the development of these diseases as a result of translocation – the migration of microbial products through the mucosal barrier.
- Bacterial translocation has been implicated in the systemic pathophysiology of diseases such as type II diabetes, systemic inflammatory responses and atherosclerosis.
- Changes in the ratio of inflammatory to suppressive cells in the gut can compromise gut mucosal integrity and cause “leakage” of microbial products into circulation
The Microbiome and Human Immunodeficiency Virus (HIV-1)
- Breakdowns in host-microbial mutualism in the intestine can also occur in a wide array of immunodeficiency-related conditions
- HIV infection and common variable immunodeficiency (CVID) are examples of acquired or congenital immunodeficiencies that include an enteropathy (chronic intestinal inflammation).
- During acute HIV-1 infection, over 50% of the CD4+ T cells are preferentially depleted from the lamina propria because of direct infection and activated-induced cell death
- HIV infection also results in relative depletion of TH17 cells.
- Experimental models with Rhesus macaques infected with the homologous retrovirus simian immunodeficiency virus (SIV) as well as HIV-infected humans who have undergone TH17 depletion exhibit increased translocation of bacteria and viruses.
- Studies of patients infected with HIV-1, linked with models of Cd4−/− mice, provide evidence that CD4+ T cells are required for anatomical containment of the microbiota
- Thus, depletion of these cells during HIV infection leads to a loss of direct tolerogenic responses to microbial signals, rather than driving shifts in composition of the microbiota.